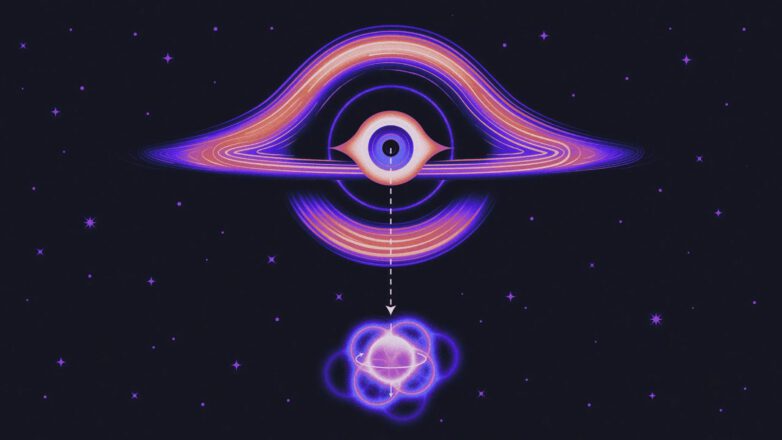
At Princeton University in the early 1970s, the celebrated theoretical physicist John Wheeler could be spotted in seminars or impromptu hallway discussions drawing a big âU.â The letterâs left tip represented the beginning of the universe, where everything was uncertain and all quantum possibilities were happening at the same time. The letterâs right tip, sometimes adorned with an eye, depicted an observer looking back in time, thus bringing the left side of the U into existence.
In this âparticipatory universe,â as Wheeler called it, the cosmos expanded and cooled around the U, forming structures and eventually creating observers, like humans and measuring apparatus. By looking back to the early universe, these observers somehow made it real.
âHe would say things like âNo phenomenon is a true phenomenon until itâs an observed phenomenon,ââ said Robert M. Wald, a theoretical physicist at the University of Chicago who was Wheelerâs doctoral student at the time.
Now, by studying how quantum theory behaves on the horizon of a black hole, Wald and his collaborators have calculated a new effect that is suggestive of Wheelerâs participatory universe. The mere presence of a black hole, theyâve found, is enough to turn a particleâs hazy âsuperpositionââthe state of being in multiple potential statesâinto a well-defined reality. âIt evokes the idea that these black hole horizons are watching,â said coauthor Gautam Satishchandran, a theoretical physicist at Princeton.
View more
John Wheelerâs âparticipatory universeâ suggests that observers make the universe real.
âWhat we have found might be a quantum mechanical realization of [the participatory universe], but where space-time itself plays the role of the observer,â said Daine Danielson, the third author, also at Chicago.
Theorists are now debating what to read into these watchful black holes. âThis seems to be telling us something deep about the way gravity influences measurement in quantum mechanics,â said Sam Gralla, a theoretical astrophysicist at the University of Arizona. But whether this will prove useful for researchers inching toward a complete theory of quantum gravity is still anyoneâs guess.
The effect is one of many uncovered in the past decade by physicists studying what happens when quantum theory is combined with gravity at low energies. For example, theorists have had great success thinking about Hawking radiation, which causes black holes to slowly evaporate. âSubtle effects that we hadnât really noticed before give us constraints from which we can glean clues about how to go up toward quantum gravity,â said Alex Lupsasca, a theoretical physicist at Vanderbilt University who was not involved in the new research.
These observant black holes seem to produce an effect thatâs âvery arresting,â Lupsasca said, âbecause it feels like somehow itâs deep.â
Black Holes and Superpositions
To understand how a black hole could observe the universe, start small. Consider the classic double-slit experiment, in which quantum particles are fired toward two slits in a barrier. Those that pass through are then detected by a screen on the other side.
At first, each traveling particle seems to appear at random on the screen. But as more particles pass through the slits, a pattern of light and dark stripes emerges. This pattern suggests that each particle behaves like waves that pass through both slits at once. The bands result from the peaks and troughs of the waves either adding together or canceling one another outâa phenomenon called interference.
Now add a detector to measure which of the two slits the particle passes through. The pattern of light and dark stripes will disappear. The act of observation changes the state of the particleâits wavelike nature disappears entirely. Physicists say that the information gained by the detection apparatus âdecoheresâ the quantum possibilities into a definite reality.
Importantly, your detector doesnât have to be close to the slits to figure out which path the particle took. A charged particle, for example, emits a long-range electric field that might have slightly different strengths depending on whether it went through the right-hand or left-hand slit. Measuring this field from far away will still allow you to gather information about which path the particle took and will thus cause decoherence.
In 2021, Wald, Satishchandran, and Danielson were exploring a paradox brought about when hypothetical observers gather information in this way. They imagined an experimenter called Alice who creates a particle in a superposition. At a later time, she looks for an interference pattern. The particle will only exhibit interference if it hasnât become too entangled with any outside system while Alice observes it.
Then along comes Bob, who is attempting to measure the particleâs position from far away by measuring the particleâs long-range fields. According to the rules of causality, Bob shouldnât be able to influence the outcome of Aliceâs experiment, since the experiment should be over by the time the signals from Bob get to Alice. However, by the rules of quantum mechanics, if Bob does successfully measure the particle, it will become entangled with him, and Alice wonât see an interference pattern.
The trio rigorously calculated that the amount of decoherence due to Bobâs actions is always less than the decoherence that Alice would naturally cause by the radiation she emits (which also becomes entangled with the particle). So Bob could never decohere Aliceâs experiment, because she would already have decohered it herself. Although an earlier version of this paradox was resolved in 2018 with a back-of-the-envelope calculation by Wald and a different team of researchers, Danielson took it one step further.
He posed a thought experiment to his collaborators: âWhy canât I put [Bobâs] detector behind a black hole?â In such a setup, a particle in a superposition outside the event horizon will emanate fields that cross over the horizon and get detected by Bob on the other side, within the black hole. The detector gains information about the particle, but as the event horizon is a âone-way ticket,â no information can cross back over, Danielson said. âBob cannot influence Alice from inside of the black hole, so the same decoherence must occur without Bob,â the team wrote in an email to Quanta. The black hole itself must decohere the superposition.
âIn the more poetic language of the participatory universe, it is as if the horizon watches superpositions,â Danielson said.
Using this insight, they set about working on an exact calculation of how quantum superpositions are affected by the black holeâs space-time. In a paper published on the preprint server Arxiv.org in January, they landed on a simple formula that describes the rate at which radiation crosses over the event horizon and so causes decoherence to occur. âThat there was an effect at all was, to me, very surprising,â Wald said.
Hair on the Horizon
The idea that event horizons gather information and cause decoherence isnât new. In 2016, Stephen Hawking, Malcolm Perry, and Andrew Strominger described how particles crossing over the event horizon could be accompanied by very low-energy radiation that records information about these particles. This insight was suggested as a solution to the black hole information paradox, a profound consequence of Hawkingâs earlier discovery that black holes emit radiation.
The problem was that Hawking radiation drains energy from black holes, causing them to completely evaporate over time. This process would appear to destroy any information that has fallen into the black hole. But in doing so, it would contradict a fundamental feature of quantum mechanics: that information in the universe canât be created or destroyed.
The low-energy radiation proposed by the trio would get around this by allowing some information to be distributed in a halo around the black hole and escape. The researchers called the information-rich halo âsoft hair.â
Wald, Satishchandran, and Danielson were not investigating the black hole information paradox. But their work makes use of soft hair. Specifically, they showed that soft hair is created not only when particles fall across a horizon, but when particles outside a black hole merely move to a different location. Any quantum superposition outside will become entangled with soft hair on the horizon, giving rise to the decoherence effect they identified. In this way the superposition is recorded as a kind of âmemoryâ on the horizon.
The calculation is a âconcrete realization of soft hair,â said Daniel Carney, a theoretical physicist at Lawrence Berkeley National Laboratory. âItâs a cool paper. It could be a very useful construction for trying to make that idea work in detail.â
But to Carney and several other theorists working at the forefront of quantum gravity research, this decoherence effect isnât all that surprising. The long-range nature of the electromagnetic force and gravity means that âitâs hard to keep anything isolated from the rest of the universe,â said Daniel Harlow, a theoretical physicist at the Massachusetts Institute of Technology.
Total Decoherence
The authors argue that there is something uniquely âinsidiousâ about this kind of decoherence. Usually, physicists can control decoherence by shielding their experiment from the outside environment. A vacuum, for example, removes the influence of nearby gas molecules. But nothing can shield gravity, so thereâs no way to insulate an experiment from gravityâs long-range influence. âEventually, every superposition will be completely decohered,â Satishchandran said. âThereâs no way of getting around it.â
The authors therefore regard black hole horizons as taking a more active role in decoherence than was previously known. âThe geometry of the universe itself, as opposed to the matter within it, is responsible for the decoherence,â they wrote in an email to Quanta.
Carney disputes this interpretation, saying that the new decoherence effect can also be understood as a consequence of electromagnetic or gravitational fields, in combination with rules set by causality. And unlike Hawking radiation, where the black hole horizon changes over time, in this case the horizon âhas no dynamics whatsoever,â Carney said. âThe horizon doesnât do anything, per se; I would not use that language.â
To not violate causality, superpositions outside the black hole must be decohered at the maximum possible rate that a hypothetical observer inside the black hole could be collecting information about them. âIt seems to be pointing toward some new principle about gravity, measurement, and quantum mechanics,â Gralla said. âYou donât expect that to happen more than 100 years after gravity and quantum mechanics were formulated.â
Intriguingly, this kind of decoherence will occur anywhere there is a horizon that only allows information to travel in one direction, creating the potential for causality paradoxes. The edge of the known universe, called the cosmological horizon, is another example. Or consider the âRindler horizon,â which forms behind an observer who continuously accelerates and approaches the speed of light, so that light rays can no longer catch up with them. All of these âKilling horizonsâ (named after the late-19th- and early-20th-century German mathematician Wilhelm Killing) cause quantum superpositions to decohere. âThese horizons are really watching you in exactly the same way,â Satishchandran said.
Exactly what it means for the edge of the known universe to watch everything inside the universe isnât entirely clear. âWe donât understand the cosmological horizon,â Lupsasca said. âItâs super fascinating, but way harder than black holes.â
In any case, by posing thought experiments like this, where gravity and quantum theory collide, physicists hope to learn about the behavior of a unified theory. âThis is likely giving us some more clues about quantum gravity,â Wald said. For example, the new effect may help theorists understand how entanglement is related to space-time.
âThese effects have to be part of the final story of quantum gravity,â Lupsasca said. âNow, are they going to be a crucial clue along the way to gleaning insight into that theory? Itâs worth investigating.â
The Participatory Universe
As scientists continue to learn about decoherence in all its forms, Wheelerâs concept of the participatory universe is becoming clearer, Danielson said. All particles in the universe, it seems, are in a subtle superposition until they are observed. Definiteness emerges through interactions. âThatâs kind of what, I think, Wheeler had in mind,â Danielson said.
And the finding that black holes and other Killing horizons observe everything, all the time, âwhether you like it or not,â is âmore evocativeâ of the participatory universe than the other types of decoherence are, the authors said.
Not everyone is ready to buy Wheelerâs philosophy on a grand scale. âThe idea that the universe observes itself? That sounds a little Jedi for me,â said Lupsasca, who nevertheless agrees that âeverything is observing itself all the time through interactions.â
âPoetically, you could think of it that way,â Carney said. âPersonally, Iâd just say that the presence of the horizon means that the fields living around it are going to get stuck on the horizon in a really interesting way.â
When Wheeler first drew the âbig Uâ when Wald was a student in the 1970s, Wald didnât think much of it. âWheelerâs idea struck me as not that solidly grounded,â he said.
And now? âA lot of the stuff he did was enthusiasm and some vague ideas which later turned out to be really on the mark,â Wald said, noting that Wheeler anticipated Hawking radiation long before the effect was calculated.
âHe saw himself as holding out a lamp light to illuminate possible paths for other people to follow.â
Original story reprinted with permission from Quanta Magazine, an editorially independent publication of the Simons Foundation whose mission is to enhance public understanding of science by covering research developments and trends in mathematics and the physical and life sciences.
#Researchers #Argue #Black #Holes #Destroy #Quantum #States